Research in the Niell lab is focused on understanding how the visual system performs the computations underlying our perception of the natural world, and how this enables different types of behaviors. We are pursuing this in three main branches of research : Natural visual behavior, Visual processing and brain states, and Neural circuits for vision in the octopus.
Natural visual behavior
In the natural world, we use our vision to analyze complex scenes and enable a wide range of specific behaviors, many of which require moving through the environment. However, in practice most studies of vision are performed in stationary subjects performing artificial tasks (e.g. pressing a lever) in response to simple stimuli (e.g. gratings). These types of paradigms therefore do not tap into much of what the brain evolved to do, and therefore likely provide a limited view of sensory processing (Parker et al 2020; Skyberg and Niell 2024). In order to address this, we have developed paradigms to study visual processing in ethological behaviors. Our primary task is prey capture – we have shown that laboratory mice will pursue and capture insect prey, and they use vision to do this. This prey capture paradigm has provided an approach to measure visual function in mice (Hoy et al 2016), to probe the role of cell types in the visual system (Hoy et al 2019), and to study active sensing and eye movements (Michaiel et al 2020). Recently, we have begun to expand this approach to include a task to measure depth perception by judging distance across a gap (Parker et al 2021), and methods to measure visual coding from the perspective of freely moving mice (Parker et al 2022).
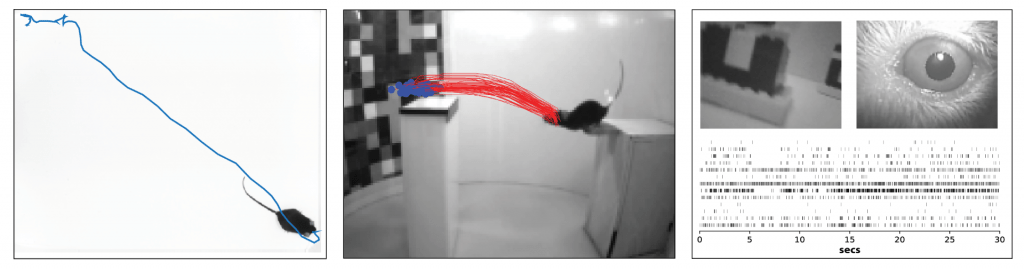
Left: Trajectory of a mouse pursuing cricket during prey capture. Middle: Multiple trajectories showing accuracy of a mouse jumping across a gap based on visual cues. Right: Simultaneous measurement of neural activity, visual input, and eye position in a freely moving mouse.
Visual processing and brain states
Visual processing is dynamic, reflecting changes in internal state, the environment, and other contextual factors, which together shape how we see the world. We investigate this in the mouse, which provides a powerful genetic model for studying neural circuits of the mammalian visual system (Niell & Stryker 2008; Huberman & Niell 2011). In early studies, we discovered dramatic differences in visual function between when an animal is moving versus sitting still (Niell & Stryker 2010; Lee et al 2014). This likely represents both global shifts in brain state during movement, and specific signals that integrate movement with the visual input (Parker et al 2020). Likewise, performing a visual task shifts how visual information is processed, relative to passively viewing the same images (Wekselblatt et al 2016; Wekselblatt et al 2019). Finally, hallucinogenic serotonergic agonists cause dramatic alterations in perception and cognition, with corresponding changes in visual evoked activity in cortex (Michaiel et al 2019). Determining how these types of changes in brain state impact visual processing is essential to a complete understanding of how vision works in the complexity of our ongoing lives.
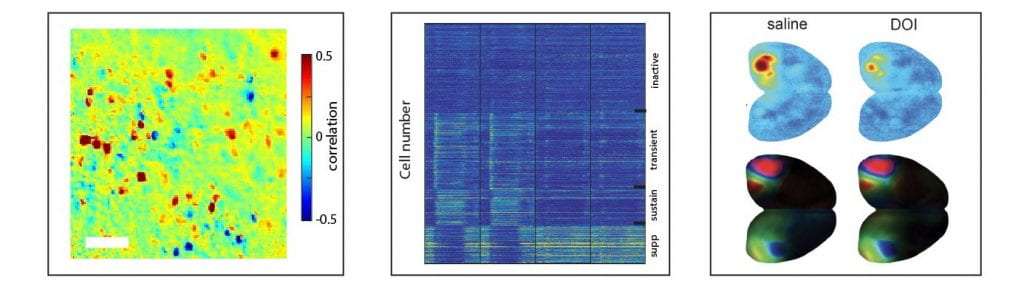
Left: 2-photon mapping of neurons that encode locomotion. Middle: Clustering of response types in V1 based on temporal dynamics during a perceptual task. Right: Effect of a 5HT-2A agonist (DOI) on visual responsiveness (top) and spatial organization (bottom).
Neural circuits for vision in the octopus
Studies of the mouse visual system are valuable due to the similarity across mammalian brains, including humans, combined with the abundance of genetic tools to study neural function in the mouse. On the other hand, the octopus represents a fundamentally different approach to studying vision (reviewed in Pungor & Niell, 2023). Octopuses have the largest and most complex brains outside of vertebrates, with a highly developed visual system – indeed, an adult octopus brain is roughly the same size as a mouse’s, but 2/3 of it is devoted to vision. Furthermore, the octopus has a range of advanced visually-driven behaviors, from prey capture to camouflage. However, it is largely unknown how this “alien” brain processes visual information, as there has never been a measurement of receptive fields in the central visual system of a cephalopod. We are currently using two-photon calcium imaging to measure neural activity in the octopus optic lobe, to define the visual features that are encoded (Pungor et al, 2023). In parallel, we are using single-cell transcriptomics to define the cell types and circuitry of the optic lobe (Songco-Casey et al, 2022). Our goal is to combine these to an understanding of how the computations performed by the octopus visual system and relate these to their repertoire of visual behaviors.